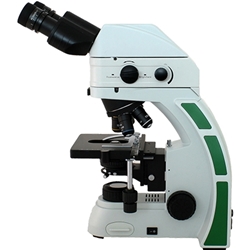
What Is Fluorescence Microscopy?
Fluorescence microscopy is a type of optical microscopy that uses fluorescence to study the properties of biological and non-biological specimens. In this imaging technique, specimens are labeled with fluorescent dyes or proteins that emit light of a specific color when illuminated with light of a different color. This emitted light is then detected and used to create detailed, high-contrast images of the specimen.
How Does Fluorescent Microscopy Work?
Fluorescence microscopy is an advanced optical technique that uses fluorescence to visualize and study specimens at the microscopic level. Here's a step-by-step explanation of how fluorescent microscopy works:
- Fluorescent Labels: The process begins with the application of fluorescent labels to the specimen. These labels are often specific to certain structures or molecules within the specimen. Fluorophores, such as fluorescent dyes or proteins, are commonly used for labeling.
- Excitation: The specimen is then exposed to light of a specific wavelength, known as the excitation wavelength. This light excites the fluorescent labels, causing them to absorb photons and move to a higher energy state.
- Emission: As the excited fluorescent labels return to their ground state, they release excess energy in the form of light. This emitted light, known as fluorescence, occurs at a longer wavelength than the excitation light.
- Filtering: Specialized filters are employed to separate the emitted fluorescence from the excitation light. These filters allow only the fluorescence to reach the detector while blocking the excitation light.
- Detection: The emitted fluorescence is detected by a camera or photodetector. The signal is then translated into an image, creating a visual representation of the labeled structures within the specimen.
- Image Formation: The collected fluorescence signals are used to construct detailed images of the specimen. Various imaging techniques, such as confocal microscopy or total internal reflection fluorescence (TIRF) microscopy, can be employed to enhance resolution and contrast.
Which Applications Are Fluorescence Microscopy Used For?
Fluorescence microscopy is a versatile and widely used technique in various scientific fields, particularly in biology and medicine. It is employed for a range of applications due to its ability to selectively visualize and study specific structures, molecules, or processes within cells and tissues. Here are some common uses of fluorescence microscopy:
- Cellular Imaging: Fluorescence microscopy is extensively used for imaging cells and cellular structures. It allows researchers to visualize cellular components such as the nucleus, cytoskeleton, and organelles with high specificity.
- Protein Localization and Expression: Fluorescent proteins and dyes are used to label and track proteins within cells. This enables the study of protein localization, expression levels, and dynamic changes in real-time.
- Molecular and Cellular Biology: Researchers use fluorescence microscopy to study cellular processes, including cell division, migration, and apoptosis. It provides insights into the mechanisms underlying various biological phenomena.
- Immunofluorescence Staining: Fluorescence microscopy is widely employed in immunofluorescence techniques, where antibodies labeled with fluorophores are used to detect and visualize specific antigens in tissues.
- Live Cell Imaging: Fluorescence microscopy allows for the observation of live cells over time. This is crucial for studying dynamic processes, cellular behavior, and responses to stimuli.
- Fluorescence In Situ Hybridization (FISH): FISH is a molecular biology technique that utilizes fluorescence probes to detect and localize specific nucleic acid sequences within cells or tissues. It is commonly used for genetic and genomic studies.
- Neuroscience Research: Fluorescence microscopy is applied in neuroscience to study neuronal structures, synaptic activity, and intracellular signaling. It aids in understanding the complexities of the nervous system.
- Microorganism Detection: Fluorescence microscopy is used in microbiology to detect and identify microorganisms, including bacteria, viruses, and fungi. Fluorescent labeling enhances the visibility of these microorganisms in complex samples.
- Flow Cytometry: Fluorescence microscopy is integrated into flow cytometry, a technique used for the analysis and sorting of individual cells based on their fluorescence properties. This is valuable in cell biology, immunology, and clinical diagnostics.
- Drug Discovery: In pharmaceutical research, fluorescence microscopy is employed to study the effects of drugs on cells and tissues. It helps assess drug efficacy, toxicity, and mechanisms of action.
The versatility and specificity of fluorescence microscopy make it an indispensable tool for researchers and clinicians, contributing to advancements in various scientific disciplines and medical applications.
Which Light Is Used in Fluorescence Microscopy?
Fluorescence microscopy uses specific wavelengths of light to excite fluorophores and induce fluorescence. The process involves the following key steps:
- Excitation: Fluorophores are molecules that can absorb light at one wavelength and emit light at a longer wavelength. In fluorescence microscopy, the specimen is stained or labeled with fluorophores. The light used for excitation is of a shorter wavelength (higher energy) than the emitted fluorescence.
- Fluorescence Emission: When the specimen is exposed to the excitation light, the fluorophores absorb the photons and become excited to a higher energy state. As the fluorophores return to their ground state, they emit fluorescence, which is detected and captured for imaging.
- Filter Systems: Fluorescence microscopes are equipped with specific filter sets to separate the excitation and emission light. A dichroic mirror or beamsplitter reflects the excitation light toward the specimen while allowing the emitted fluorescence to pass through to the detector.
The choice of excitation light depends on the properties of the fluorophores used in the sample. Commonly used light sources for fluorescence microscopy include:
- Mercury Arc Lamps: Traditional fluorescence microscopes often use mercury arc lamps as a broad-spectrum light source. These lamps emit light across a range of wavelengths, suitable for exciting a variety of fluorophores.
- Xenon Arc Lamps: Xenon arc lamps are another type of broad-spectrum light source used in fluorescence microscopy. They offer a more stable and continuous spectrum compared to mercury lamps.
- LEDs (Light-Emitting Diodes): Light-emitting diodes have become increasingly popular as light sources for fluorescence microscopy. LEDs are available in various wavelengths, providing flexibility in choosing excitation wavelengths. They are energy-efficient and have a longer lifespan than traditional lamps.
- Laser Light Sources: Lasers are highly focused and monochromatic light sources. They are used when precise control over excitation wavelengths is required. Laser scanning confocal microscopes often incorporate lasers.
The selection of the excitation light source depends on factors such as the fluorophores used, the desired excitation wavelengths, and the specific imaging requirements. Researchers choose the appropriate light source to optimize the sensitivity and specificity of fluorescence imaging for their samples.
Fluorescence Microscopy Principle
Fluorescence microscopy operates on the principle of using fluorophores, or fluorescent molecules, to selectively label specific structures or molecules within a specimen. The key principles of fluorescence microscopy include:
Fluorophore Absorption and Excitation:
- Fluorophores are molecules that can absorb light at a specific wavelength and then emit light at a longer wavelength.
- The specimen is treated with fluorophores, either through staining or labeling with fluorescent markers.
Excitation Light:
- The microscope exposes the specimen to light of a specific wavelength, known as the excitation wavelength.
- This light is typically of higher energy than the emitted fluorescence.
Energy Transfer and Fluorescence Emission:
- When the fluorophores absorb photons of the excitation light, they become excited to a higher energy state.
- As the fluorophores return to their ground state, they release excess energy in the form of fluorescence, emitting light at a longer wavelength.
Specific Wavelengths:
- Each fluorophore has characteristic excitation and emission spectra, defining the wavelengths at which it absorbs and emits light.
- By selecting appropriate fluorophores and corresponding excitation wavelengths, specific structures or molecules in the specimen can be targeted.
Filter Systems:
- Fluorescence microscopes are equipped with optical filters to separate the excitation and emission light.
- A dichroic mirror or beamsplitter reflects the excitation light towards the specimen while allowing the emitted fluorescence to pass through to the detector.
Image Detection:
- The emitted fluorescence is captured by a detector, such as a camera or photomultiplier tube.
- The resulting image highlights the fluorescently labeled structures in the specimen.
Fluorescence microscopy is widely used in biological research and medical diagnostics due to its ability to visualize specific cellular structures, proteins, or other biomolecules with high sensitivity and specificity. It enables researchers to study the spatial distribution and dynamics of fluorescently labeled components within living or fixed specimens.
Advantages And Disadvantages of Fluorescence Microscopy
Advantages of Fluorescence Microscopy
High Sensitivity and Specificity:
- Fluorescence microscopy provides high sensitivity, allowing the detection of low concentrations of fluorescently labeled molecules.
- Specificity is enhanced through the use of fluorophores that selectively bind to target structures.
Multiplexing Capability:
- Multiple fluorophores with distinct emission spectra can be used simultaneously, enabling the observation of multiple targets in a single sample.
Live-Cell Imaging:
- Fluorescence microscopy allows real-time imaging of dynamic processes within living cells, providing insights into cellular behaviors and responses.
High Resolution:
- Fluorescence microscopes can achieve high spatial resolution, allowing the visualization of subcellular structures and details.
Quantitative Analysis:
- Fluorescence intensity can be quantified, enabling researchers to perform quantitative analyses and comparisons.
Versatility in Labeling:
- Various molecules, including proteins, nucleic acids, and small molecules, can be fluorescently labeled for visualization.
Clinical and Diagnostic Applications:
- Fluorescence microscopy is widely used in clinical diagnostics, pathology, and medical research for detecting specific biomarkers and abnormalities.
Compatibility with Molecular Probes:
- Availability of a wide range of fluorescent probes and dyes allows researchers to study diverse cellular components and processes.
Disadvantages of Fluorescence Microscopy
Photobleaching:
- Continuous exposure to excitation light can lead to the fading or photobleaching of fluorophores, limiting the duration of imaging sessions.
Phototoxicity:
- High-intensity illumination required for excitation may induce cellular damage or alter biological processes in living samples.
Background Autofluorescence:
- Intrinsic fluorescence from biological samples or nonspecific binding of fluorophores can contribute to background signals, reducing image contrast.
Limited Tissue Penetration:
- Fluorescence signals may be attenuated in thick tissues due to light scattering and absorption, limiting penetration depth.
Equipment Cost and Complexity:
- High-quality fluorescence microscopy equipment can be expensive, and the techniques may require specialized training.
Artifact Generation:
- Sample preparation and labeling procedures may introduce artifacts, affecting the interpretation of results.
Limited Resolution in Deep Tissues:
- Achieving high resolution in deep tissues is challenging due to light scattering, limiting the application in thick specimens.
Limited to Fluorescent Targets:
- Only structures labeled with fluorophores are visible, and non-fluorescent components remain undetected.
While fluorescence microscopy offers numerous advantages, researchers should be mindful of its limitations and consider factors such as photobleaching, phototoxicity, and background autofluorescence when designing experiments.
Types Of Fluorescence Microscopy
Widefield Fluorescence Microscopy:
- Principle: Excitation light illuminates the entire sample, and emitted fluorescence is observed through the microscope objective.
- Application: Fast imaging, live-cell studies, and general fluorescence observations.
Confocal Fluorescence Microscopy:
- Principle: Uses a pinhole to eliminate out-of-focus light, producing optical sections and 3D reconstructions.
- Application: Improved resolution, reduced background, and optical sectioning for detailed imaging.
Two-Photon Fluorescence Microscopy:
- Principle: Two photons of lower energy simultaneously excite a fluorophore, enabling deeper tissue penetration.
- Application: Deep-tissue imaging with reduced photodamage.
Total Internal Reflection Fluorescence Microscopy (TIRF):
- Principle: Excitation occurs at the interface of two media, allowing selective illumination of a thin layer near the sample surface.
- Application: High-contrast imaging of cell membrane processes, reduced photodamage.
Structured Illumination Microscopy (SIM):
- Principle: Patterned light illuminates the sample, increasing resolution beyond the diffraction limit.
- Application: Enhanced resolution in live-cell imaging.
Stimulated Emission Depletion Microscopy (STED):
- Principle: Uses a depletion beam to reduce the size of the excitation spot, achieving super-resolution.
- Application: Nanoscale imaging with improved spatial resolution.
Fluorescence Resonance Energy Transfer (FRET) Microscopy:
- Principle: Measures energy transfer between two fluorophores in close proximity, indicating molecular interactions.
- Application: Studying protein-protein interactions, conformational changes.
Fluorescence Lifetime Imaging Microscopy (FLIM):
- Principle: Measures the fluorescence decay time to study molecular interactions and probe microenvironment.
- Application: Quantitative analysis of molecular dynamics, identifying fluorophore environments.
Fluorescence Correlation Spectroscopy (FCS):
- Principle: Analyzes fluctuations in fluorescence intensity to study molecular diffusion and interactions.
- Application: Quantitative analysis of molecular mobility, concentration, and binding.
Single-Molecule Fluorescence Microscopy:
- Principle: Detects signals from individual fluorophores, allowing the study of single molecules.
- Application: Investigating molecular behavior at the single-molecule level.
Choosing the appropriate fluorescence microscopy technique depends on the specific research objectives, sample characteristics, and desired imaging capabilities. Each method offers unique advantages and is tailored to address particular experimental requirements.
What Does PPT Stand for In Fluorescence Microscopy
In fluorescence microscopy, "PPT" often stands for "Photobleaching Protection Technology." Photobleaching is a phenomenon where the fluorophores (fluorescent molecules) used to label specimens lose their ability to fluoresce over time due to exposure to light. Photobleaching Protection Technology is designed to minimize or mitigate this effect, allowing for longer and more stable fluorescence imaging.
This technology may involve various methods to reduce photobleaching, such as optimizing the illumination conditions, adjusting the duration and intensity of light exposure, or using chemical additives that help protect fluorophores from degradation. The goal is to extend the time during which fluorescence can be observed without significant loss of signal intensity.
Check out our Fluorescence Microscope Cameras