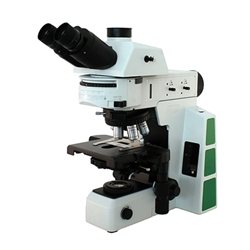
Epi-Fluorescence & Fluorescence Microscopes
In the field of microscopy, there is a vast array of optical technology available to researchers. Among these various technologies, the epi-fluorescence microscope is one of the most widely used optical tools. Epi-fluorescence microscopy can be combined with high quality Japanese optics and a variety of filter cubes to provide different levels of resolution. This type of compound microscope can also be paired with USB digital CCD cameras for image capture. The software included with these cameras allows for not only image capture, but also measurement, stacking and combining images captured with different filter sets into one image, among numerous other features. These additional technologies offer unique methods for viewing the specimen.
What is Epifluorescence Microscopy?
Epifluorescence, also known as epi-illumination or epifluorescence microscopy, refers to a specific technique used to observe fluorescently labeled samples under a microscope. In epifluorescence microscopy, the excitation light is directed onto the sample from above (epi-illumination) and the emitted fluorescence is collected through the same objective lens. This configuration allows researchers to visualize the fluorescently labeled components within the sample.
What is a Fluorescence Microscope?
A fluorescence microscope is a specialized optical instrument used to visualize and study biological or material samples that exhibit fluorescence properties. Fluorescence is a phenomenon where certain molecules, known as fluorophores, absorb light at a specific wavelength and then emit light at a longer wavelength. This emitted light, or fluorescence, is typically of a different color than the excitation light, allowing researchers to distinguish specific components within a sample.
The primary principle behind fluorescence microscopy involves labeling the target molecules with fluorescent markers, also known as fluorochromes or dyes. These markers are chosen for their ability to bind selectively to certain molecules of interest, such as proteins, DNA, or cellular structures. Once the sample is labeled, it can be illuminated with light of a specific wavelength that matches the excitation spectrum of the fluorophores.
What is Fluorescence Microscopy?
Fluorescence microscopy is an advanced imaging technique that harnesses the unique properties of fluorescence to reveal intricate details within biological and material samples at the microscopic level. It enables scientists to visualize specific molecules, structures, and interactions that are often challenging or impossible to observe using conventional microscopy methods.
Fluorescence microscopy relies on the phenomenon of fluorescence – the emission of light by certain molecules when they absorb light of a particular wavelength. Fluorophores, specialized molecules with fluorescent properties, are employed to label specific components within the sample. These components could include proteins, DNA, cellular organelles, or other molecules of interest.
The process of fluorescence microscopy can be broken down into several key steps:
Labeling: The first step involves attaching fluorophores to the molecules of interest. This is achieved by using antibodies, dyes, or other markers that have an affinity for the target molecules. When the fluorophores bind to their targets, they effectively tag these molecules for visualization.
Excitation: Once the sample is labeled, it is exposed to a light source that emits excitation light at a specific wavelength. This light excites the fluorophores, causing them to absorb energy and transition to a higher energy state.
Emission: After absorbing energy, the fluorophores return to their lower energy state by emitting light. This emitted light, known as fluorescence, typically has a longer wavelength than the excitation light. The emitted fluorescence carries information about the location and presence of the labeled molecules.
Detection: The emitted fluorescence light is collected by the microscope's objective lens. It passes through filters and dichroic mirrors that isolate the fluorescence signal from the excitation light. The collected fluorescence is then directed to a detector, such as a camera or a photomultiplier tube, which converts the light signal into an electrical signal.
Image Formation: The electrical signal is processed and translated into a visual image on a computer screen or other display. The resulting image represents the distribution of labeled molecules within the sample. Different colors may be assigned to different fluorophores, allowing for multicolor imaging and the simultaneous visualization of multiple components.
How Does a Fluorescence Microscope Work?
The process begins with the excitation of fluorescent molecules using a light source. When these molecules absorb the excitation light, they enter an excited state and emit longer wavelengths of light, a phenomenon known as fluorescence. Filters and dichroic mirrors then isolate the emitted light, which is subsequently magnified by lenses and captured by detectors, resulting in vivid and informative images.
Benefits of Fluorescence Microscopes
Fluorescence microscopes offer a wide range of benefits that make them indispensable tools in various scientific and research fields. Their unique capabilities provide researchers with the ability to explore and understand complex biological and material systems in ways that were once unimaginable. Here are some key benefits of fluorescence microscopes:
Enhanced Sensitivity and Specificity
Fluorescence microscopy enables researchers to achieve high levels of sensitivity and specificity when visualizing specific molecules or structures within a sample. By selectively labeling target molecules with fluorophores, scientists can focus their observations on particular components of interest.
Molecular Imaging
Fluorescence microscopes allow for the visualization of individual molecules and cellular structures, enabling researchers to study molecular interactions, protein localization, and dynamic processes in real-time. This level of detail provides insights into cellular and molecular behaviors that would be challenging to observe using other techniques.
Multicolor Imaging
Fluorescence microscopes can simultaneously visualize multiple molecules or structures by using different fluorophores that emit light at distinct wavelengths. This multicolor imaging capability enables the examination of complex interactions and spatial relationships within a sample.
Live Cell Imaging
Fluorescence microscopy is well-suited for live cell imaging, allowing researchers to observe and track dynamic processes within living cells over time. This is crucial for understanding cell behavior, division, movement, and responses to various stimuli.
Three-Dimensional Imaging
Fluorescence microscopy can provide three-dimensional information about the spatial distribution of labeled molecules within a sample. Techniques such as confocal microscopy and two-photon microscopy help researchers obtain optical sections and reconstruct three-dimensional images of complex structures.
Subcellular Resolution
With high numerical aperture objectives and advanced optical techniques, fluorescence microscopes can achieve subcellular resolution, revealing detailed structures and interactions within cellular compartments.
Clinical and Medical Applications
Fluorescence microscopy plays a significant role in medical research and diagnostics. It aids in identifying disease markers, studying the progression of diseases, and developing targeted therapies. In fields like pathology, oncology, and microbiology, fluorescence microscopy helps researchers understand disease mechanisms and cellular responses.
Materials Science and Nanotechnology
Fluorescence microscopes are used in materials science to analyze material properties at the nanoscale. They are employed in characterizing nanomaterials, studying molecular interactions on surfaces, and investigating the behavior of nanoparticles.
Photobleaching and Photoactivation Techniques
Fluorescence microscopes can also be used in advanced techniques such as photobleaching and photoactivation. These techniques provide insights into molecular dynamics, protein mobility, and cellular processes by selectively bleaching or activating specific fluorophores.
Research Advancements
The detailed information obtained from fluorescence microscopy has led to numerous scientific breakthroughs across various disciplines. It has expanded our understanding of fundamental biological processes, disease mechanisms, cellular signaling pathways, and the properties of novel materials.
What Is a Fluorescence Microscope Used For?
Fluorescence microscopes have a diverse range of applications across various scientific fields due to their unique ability to visualize specific molecules and structures within biological and material samples. Their versatility and precision make them invaluable tools for researchers, clinicians, and scientists. Here are some of the key applications of fluorescence microscopes:
Cell Biology and Immunology
Fluorescence microscopy plays a crucial role in cell biology and immunology by allowing researchers to study cellular structures, organelles, and molecular interactions. It enables the visualization of cellular processes such as mitosis, endocytosis, and cell signaling, as well as the localization of proteins and other molecules within cells.
Genetics and Molecular Biology
In genetics and molecular biology, fluorescence microscopes aid in studying DNA, RNA, and other genetic material. Researchers use fluorescent probes to track gene expression, visualize DNA replication, and study chromosomal abnormalities.
Neuroscience
Neuroscientists utilize fluorescence microscopy to investigate the complex structures and interactions within the nervous system. It enables the visualization of neuronal pathways, neurotransmitter distribution, and dynamic processes in live neurons, shedding light on brain function and neurological disorders.
Medical Research and Diagnostics
Fluorescence microscopes have vital applications in medical research and diagnostics. They are used to identify disease markers, study tissue samples, diagnose infections, and analyze the progression of diseases such as cancer. Fluorescent probes can also track the uptake and distribution of drugs within cells and tissues.
Developmental Biology
Researchers in developmental biology employ fluorescence microscopy to study embryonic development, tissue formation, and organogenesis. It allows the visualization of cell migration, differentiation, and morphogenesis during the early stages of life.
Microbiology
Fluorescence microscopy is utilized in microbiology to observe microorganisms, track bacterial pathogens, and study microbial interactions. It aids in identifying bacteria, viruses, and other pathogens in clinical samples.
Materials Science and Nanotechnology
In materials science, fluorescence microscopy is used to analyze the properties and behavior of nanomaterials and molecular assemblies. It helps researchers understand the characteristics of materials at the nanoscale and study the interactions between molecules on surfaces.
Environmental Studies
Fluorescence microscopes are employed in environmental studies to analyze samples from various ecosystems. They help researchers identify and study microorganisms, algae, and pollutants in water, soil, and air samples.
Live Cell Imaging
The ability to capture dynamic processes in real time is a hallmark of fluorescence microscopy. Live cell imaging allows researchers to track cellular behaviors, movements, and responses to stimuli, providing insights into cell physiology and behavior.
Biotechnology and Drug Development
Fluorescence microscopy is instrumental in biotechnology and drug development. It assists in the screening of potential drug compounds, monitoring cellular responses to treatments, and evaluating the efficacy of therapeutic agents.
How Is a Fluorescence Microscope Different from a Confocal Microscope?
While both fluorescence and confocal microscopes exploit fluorescence principles, they differ in their imaging techniques. Fluorescence microscopes generate images by collecting emitted fluorescence from the entire sample, offering excellent sensitivity. In contrast, confocal microscopes use a pinhole aperture to eliminate out-of-focus light, resulting in sharper optical sections and three-dimensional reconstructions.
The key components of a fluorescence microscope include:
Light Source: The microscope employs a light source that emits excitation light of the desired wavelength. Common light sources include high-intensity lamps, lasers, and light-emitting diodes (LEDs).
Excitation Filter: This filter is positioned between the light source and the sample to ensure that only the excitation wavelength reaches the specimen. It helps prevent unwanted wavelengths from interfering with the imaging process.
Objective Lens: The objective lens focuses the excitation light onto the specimen and collects the emitted fluorescence light. Its high numerical aperture allows for high-resolution imaging.
Dichroic Mirror (Beam Splitter): Placed at a 45-degree angle, this mirror reflects the excitation light towards the specimen while allowing the emitted fluorescence to pass through to the detector.
Emission Filter: This filter is located between the specimen and the detector, ensuring that only the fluorescence emitted at the desired wavelength reaches the detector. It further eliminates any remaining excitation light.
Detector: The detector captures the emitted fluorescence, converting it into an electrical signal. Common detectors include charge-coupled devices (CCDs) and photomultiplier tubes (PMTs).
Image Formation: The captured fluorescence signal is processed and transformed into a visible image on a display screen. This image reveals the spatial distribution of the labeled molecules within the sample.
Shop By
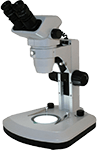
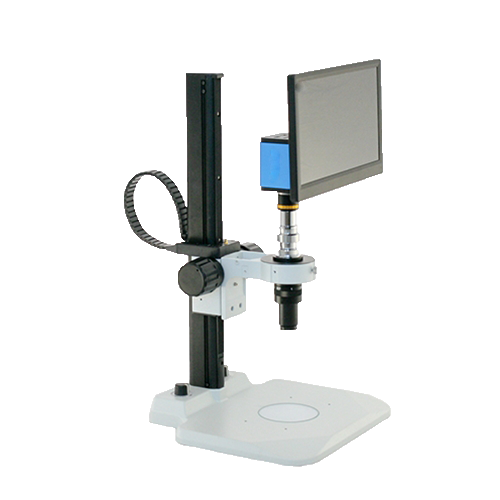